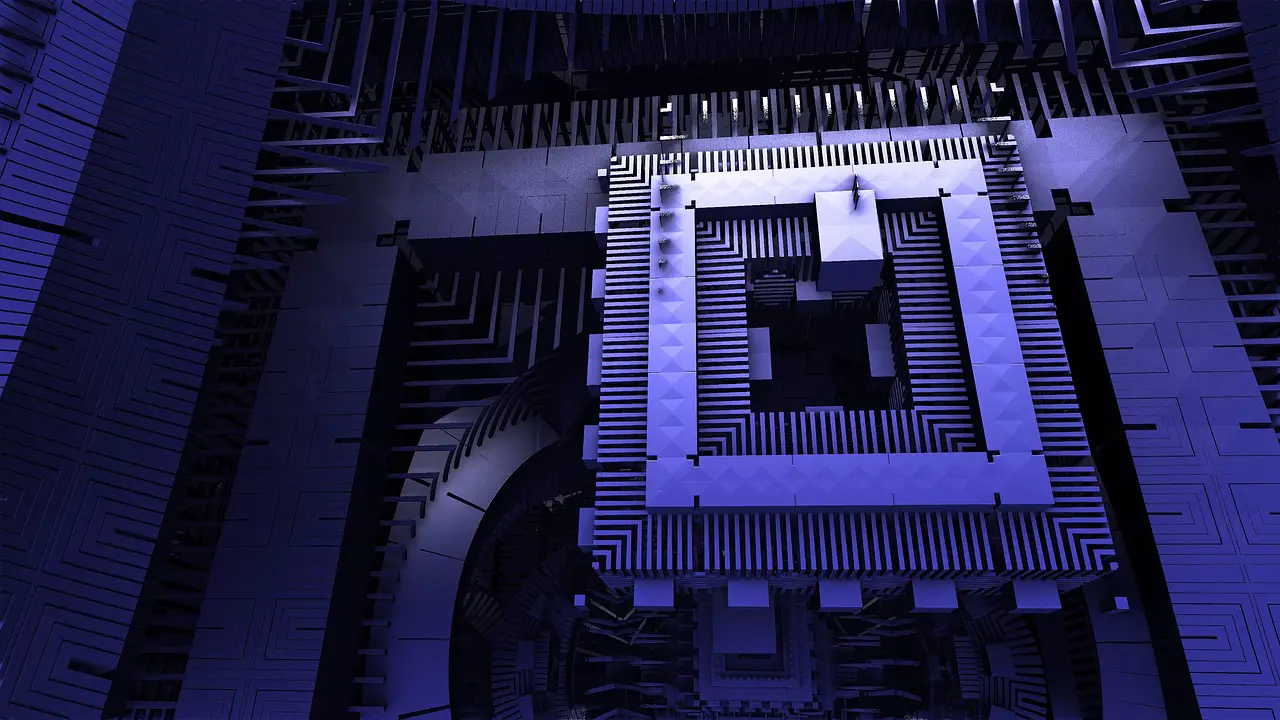
Quantum computing technologies based on superconducting qubits have garnered attention for their efficiency and scalability potential. However, for two decades, experts have grappled with a persistent issue: measurements in these systems are less accurate than theoretical predictions. A solution to this puzzle has now been found—a research team led by Marie-Frédérique Dumas from the University of Sherbrooke in Canada has developed a theoretical model that elucidates the causes of these discrepancies.
The study focused on the most common type of superconducting qubit—the transmon. To extract information about a qubit’s state, a microwave signal is passed through a resonator connected to the qubit. In analyzing the qubit-resonator system, researchers employed three distinct approaches—ranging from quantum to fully classical, where the transmon was modeled as a mechanical pendulum under external influence.
The team discovered that transmitting a microwave signal through the resonator could cause the qubit to absorb energy, propelling it to a higher state beyond its potential “well.” This process, termed “qubit ionization,” results in the loss of its defined quantum state and degrades measurement accuracy. Theoretical predictions from the three models aligned with experimental data.
According to the researchers, this new model not only clarifies the low measurement accuracy in transmon qubits but also holds promise for studying other types of superconducting qubits and enhancing readout techniques.